​
3D bacterial motion and particle tracking by digital holographic microscopy
​
​
Research Title: Study of 3D bacterial motion and biofilm formation by digital holographic microscopy.
​
Supervisor: Dr. Hangjian Ling, University of Massachusetts Dartmouth.
Goal: To observe the bacteria attachment behavior on the surfaces and compare it with the bulk motion
​
Summary: In this work, we applied Digital Holographic Microscopy (DHM) to study the 3D bacterial motion and biofilm formation. The bacterial sample is placed in a closed chamber with a thickness of 200 µm and the bacterial motion recorded at 50 frames per second continuously for 40 s at every 1 hour. The experiment begins at a low bacterial concentration of 500 cells/mm3 and run for a duration of 4 hours by which the concentration increased to 6000 cells/mm3.
​
​
​
​
​
​​
​
​
​
Figure 1. (a) Optical setup of DHM for imaging the 3D motion and growth of bacteria in a closed glass cuvette (the inner walls of the cuvette are located at z=0 and 200 µm, and bacteria are located at 0<z<200 µm); (b) a sample hologram of one bacteria located at 34 µm away from the hologram plane; (c-d) intensity distributions in the center of the bacteria in y-z plane (c) and x-y plane (d) obtained by reconstruction of the hologram shown in (b).
​
The holograms are post-processed to generate 3D trajectories of bacteria. Two strains of Shewanella sp. are studied, one showing preferential growth near the surface (i.e., creating biofilm), while the other exhibiting similar growth rates throughout the chamber (i.e., no detectable biofilm formation).
Figure 2. Sample 3D trajectories for UMDC1 (a) and UMDC19 (b) obtained at t=4 hours. The number of trajectories shown in (a) and (b) are 742 and 688 respectively.
​
​
​
​
Figure 3. Growth statistics for bacteria UMDC19 with preferential growth on solid surfaces: Spatial distributions of bacteria in the chamber at the begin ( t=0) and end ( t=4 hours) of the experiments (the two walls of the chamber are at z=0 and 200 µm); and histogram of bacteria position in z direction(right image)
​
​
​
​
​
Figure 4. Growth statistics for bacteria UMDC1 with no preferential growth on solid surfaces: Spatial distributions of bacteria in the chamber at the begin ( t=0) and end ( t=4 hours) of the experiments (the two walls of the chamber are at z=0 and 200 µm); and histogram of bacteria position in z direction(right image)
​
-
To understand which movement behavior contributes to the wall accumulation, we analyze the movement statistics. First, we compared the swimming velocities of the two bacteria species: velocity parallel to the wall & velocity perpendicular to the wall. Lastly, we compared the 3D movement trajectories of the two strains of bacteria in the bulk (50<z<150 µm) and near-wall (0<z<10 µm and 190<z<200 µm) regions. We classified the trajectories by first calculating the mean square displacement (∆r2) and then fitting ∆r2 with respective to time lag (∆t) as ∆r2=∆tK, where K is the power exponent. Based on the measured value of K, the trajectories were divided into two categories: sub-diffusive (or in-active) motion for K<1, and super-diffusive (or active) motion for K>1.We analyze and compare the velocity and mean-square-displacement (MSD) of the two strains.
​
For details, please refer to: M. Elius, K. Boyle, W.S. Chang, P. H. Moisander, H. Ling, Comparison of 3D motion of bacteria with and without
wall accumulation. Physical Review E 108 (1): 014409 (2023)
​
​
​
​
​
Particle tracking using digital holographic microscopy​​​​​​​​
​
​
Supervisor: Dr. Hangjian Ling, University of Massachusetts Dartmouth.
​
In this work, we discusses the effect of hologram plane position on the tracking of particle motions in a 3D suspension using digital holography microscopy. We compare two optical configurations where the hologram plane is either located just outside of the particle suspension or located in the middle of the suspension. In both cases, we record two axially-separated holograms using two cameras and subsequently adopt an iterative phase-retrieval approach to solve the virtual image problem. We measure the settling motions of 2 mm spheres in a 2 mm thick sample containing 300 to 1500 particles/mm3. We show that the optical setup where the hologram plane is located in the middle of the sample provides superior tracking results compared to the other, including a higher accuracy in the measurement of particle displacement and longer particle trajectories. This superior outcome is due to the less overlapping of the fringe patterns on the holograms when the separation distance between particles and hologram plane is minimized
​
​
​
​
​
​
​
​
​
​
Figure (a) Optical setup of DHM for simultaneously recording two holograms; (b-c) optical configurations where the hologram planes are located outside of the sample (b) and within the sample (c).
​
​
​
​
​Figure. (a-b) Particle trajectories obtained from the two optical configurations. The dark bots indicate the end of each trajectory. (c-e) Probability distributions of the particle displacement in the x direction (c), particle displacement in the z direction (d), and particle trajectory length (e). The data is obtained at a particle concentration of 330 particles/mm3.
​
For details, please refer to Elius & Hangjian Ling(2022)


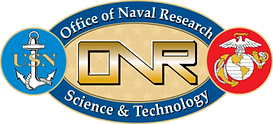
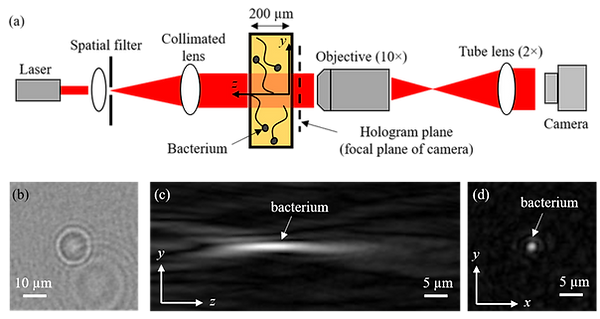


